Radio galaxies comprise the ~10% of active galactic nuclei (AGN) possessing strong collimated outflows (jets). These AGN differ from blazars, the most commonly detected VHE source, in that their jets are not aligned towards the
observer. Because of this they are rarely detected in the gamma-ray band. However, they also present opportunities for studies of the physics within jets. 3C 264 was observed by VERITAS from 2017-2019, and was discovered as a VHE emitter during an elevated flux state in 2018. It is now the fourth VHE radio galaxy known and the most distant.
The identification of an elevated flux state triggered a deep VERITAS campaign, along with a intensive multi-wavelength (MWL) follow-up effort including some of the world?s most powerful observatories (Chandra, Swift, HST, VLBA). The goal of the campaign was to identify the source of the VHE emission and/or flux enhancement within the jet. While a modestly elevated VHE and X-ray state is seen in 2018, there was no clear change in the high-resolution radio, optical or X-ray morphology. The SED for 3C 264 can be reasonably described by a synchrotron self-Compton (SSC) model typical of blazars, although it is likely a more complex model is needed to fully characterize the data. Although 3C 264 and M87 show many morphological similarities, and both are VHE detected, their SEDs aredramatically different. Qualitatively, this could be due to jet alignment where 3C 264 is more aligned.
Figure 1: VERITAS sky map of the signi ficance observed from the direction of 3C 264 during 2018. The centroid of the excess observed by VERITAS is within 2 σ of the SIMBAD position of 3C 264 (black cross). The extent of the VHE source is consistent with the VERITAS point-spread function (PSF). The PSF in this analysis is reduced from prior publications due to the use of the ITM γ-ray reconstruction (Christiansen 2017).
Figure 2: Bottom: VHE light curve measured by VERITAS. The flux was elevated in February and March of 2018. Upper limits at the 99% CL are also shown for the 2017 and 2019 observations due to the low signifi cance of the observed excesses. The flux observed in 2017-19 is indicated by the line (short dashed). The 2018 flux is indicated by the dashed line segment. Top: X-ray flux light curve measured
by Swift-XRT. The average flux from 2018-2019 is indicated by a dashed line.
Figure 3: VHE spectrum observed by VERITAS in 2018.
Figure 4: Swift-XRT spectrum and fi t for 2018 and 2019.
Figure 5: Deconvolved Chandra HRC-I image of 3C 264 observed on 04 Apr 2018. The HST image was aligned assuming the brighter component to the south is the core, and the resulting overlay of the HST contours of the jet is shown. The previously reported thermal emission associated with the host galaxy by Sun et al. (2007) is on the scales of 1.5-6". This image shows very little emission on the same scales (the green circle has a radius of 1.5"). Color scale is in counts units.
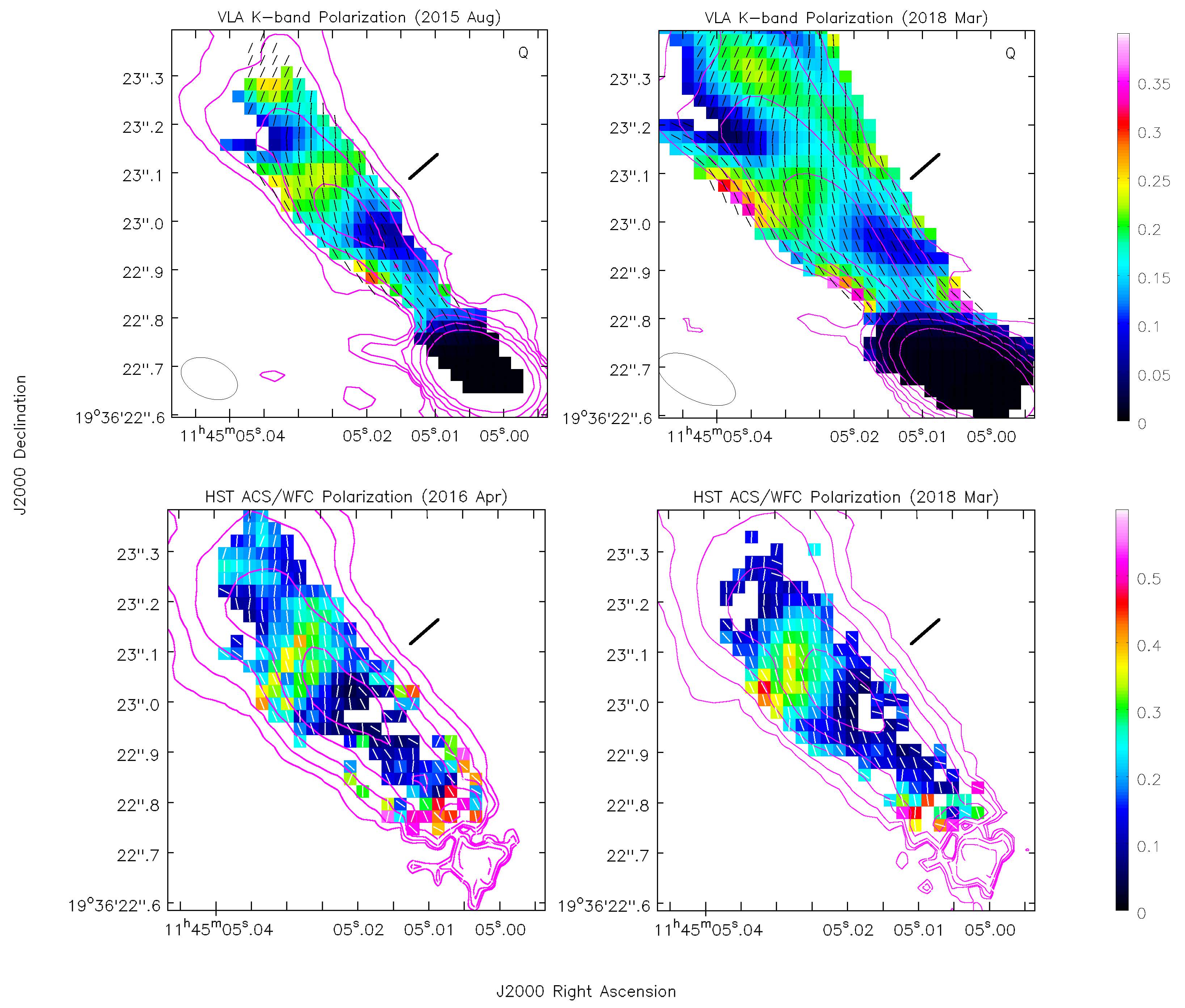
Figure 6: High-resolution radio and optical polarization images of 3C 264 from the VLA and HST. These images all show the fractional linear polarization, on a scale from 0% to 40% in the radio, and from 0% to 60% in the optical. The VLA K-band polarization is shown in August 2015 (top left) and March 2018 (top right). There is no striking di fference in the level of polarization in the jet, both showing a peak of about 22-23% polarization just downstream of the knot B/C collision region, indicated by the black line (Meyer et al. 2015). The peak of HST-optical polarization is in the same location and reaches 15-17%. It also shows similar levels in April 2016 (bottom left) and March 2018 (bottom right). The polarization values are uncorrected for the eff ect of dilution from the light from the galaxy/dust disk. In all images, the vector lines show the direction of the magnetic fi eld (90o rotation from the EVPA), and the contour lines show the flux in the corresponding Stokes-I image for each epoch/band. In the radio images, contours are drawn at 1, 2, 4, 8 and 16 times the base level of 5x 10-4 Jy. In the
optical images, the contour lines are drawn at 2, 4, 8, 16, and 32 times the base level of 1 x10-8 Jy.
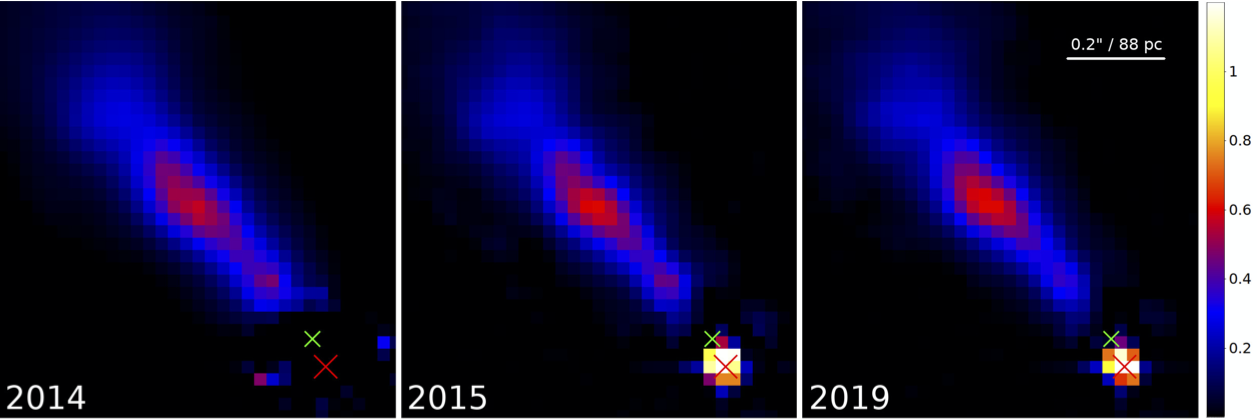
Figure 7: HST images of the kpc-scale jet in 3C 264. In all cases, the light from the galaxy and inner dust disk is modeled and subtracted. The images from May 2014 (left; ACS/WFC F606W), November 2015 (center; WFC3/UVIS F814W) and January 2019 (right; WFC3/UVIS F814W) are shown. The red cross marks the location of the central black hole, and the green cross marks the location of a bend in the jet seen in VLBA imaging. Colorbar scale shown at left is in units of μ Jy.
Figure 8: Results from VLBA images of 3C 264 taken at 5.0 GHz, 8.4 GHz, 12.1 GHz, and 15.3 GHz on 30 Mar 2018. Maps of the radio spectral index (left) and the synchrotron spectral turnover frequency (right) are shown. The VLBA images were restored with a common Gaussian beam having FWHM dimensions 3.4 x 1.5 mas at position angle -9o . The 5.0 GHz total-intensity contours are drawn at successive factors of two times the base-contour level of 0.4 mJy beam-1.
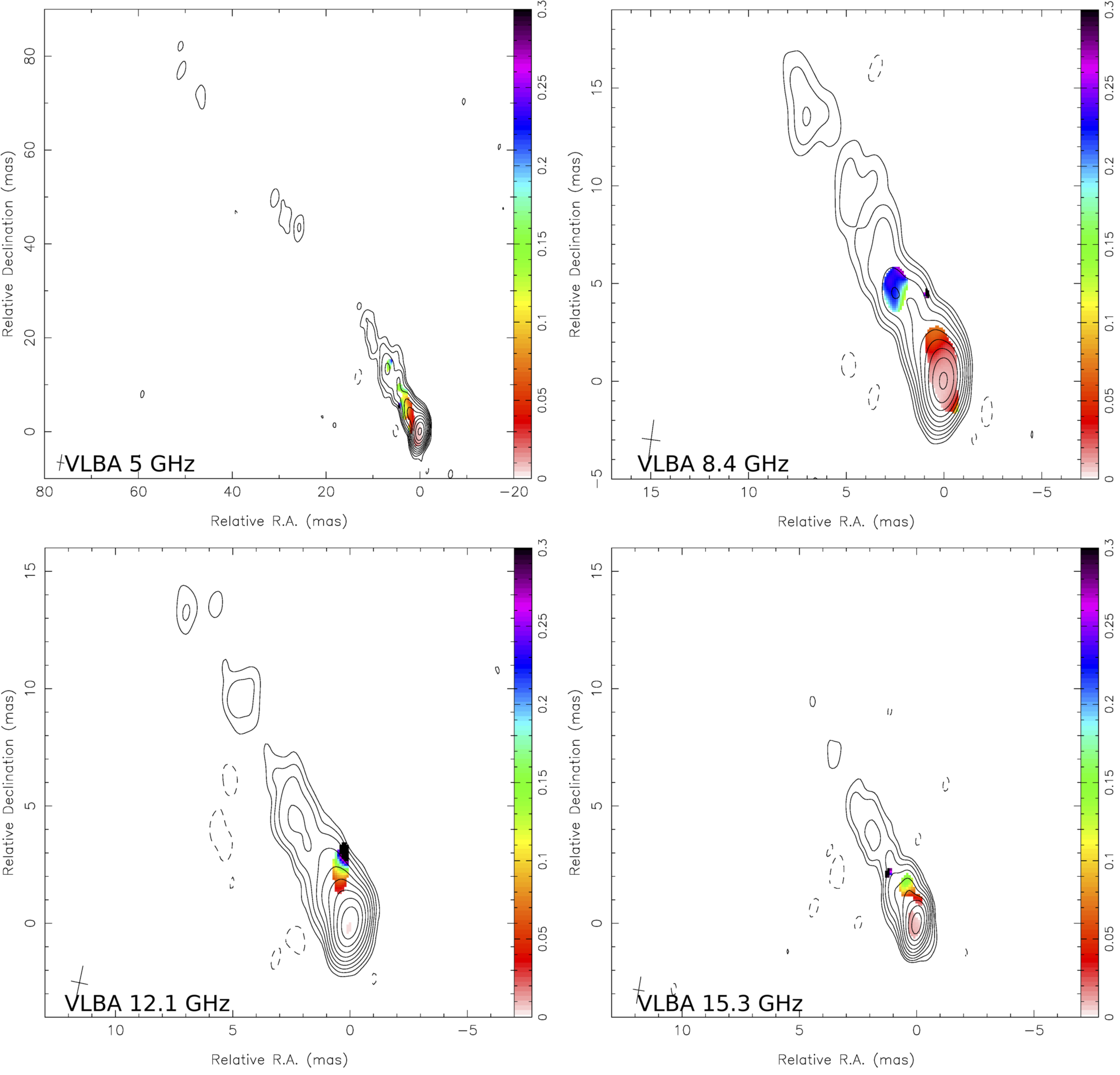
Figure 9: VLBA naturally-weighted contour maps of 3C 264 at 5.0, 8.4, 12.1 and 15.3 GHz. The fractional linear polarization is overlaid in false color for pixels with total linearly polarized intensity above 0.2, 0.45, 0.45 and 0.5 mJy beam-1 , respectively. The contours are drawn in successive factors of two times the base contour level of 0.2, 0.25, 0.29 and 0.3 mJy beam-1 . A single negative contour equal to the base contour is also drawn using dashed lines. The peak total intensity of the map is 119, 144, 132, and 116 mJy beam-1 , respectively. The dimensions and orientation of the restoring beam are indicated by a cross at the lower left of each sub-fi gure. The 5.0-GHz image (top-left) is shown on a different, larger scale.
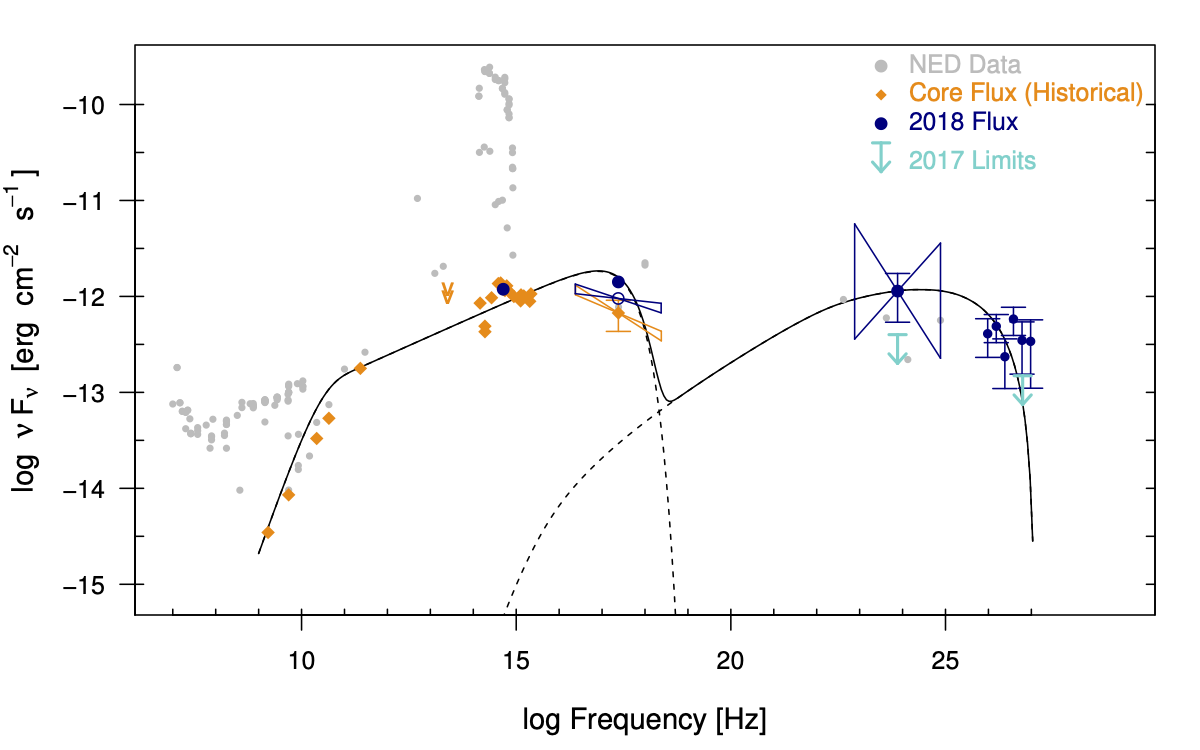
Figure 10: The broad-band SED for 3C 264. Gray points are historical fluxes from NED, where the low-frequency radio is dominated by the isotropically-emitting radio lobes and the optical by the host galaxy. Shown in orange are the isolated flux values for the core as seen by VLA, ALMA, HST, and Chandra (data taken from NED, this paper, and Perlman et al. 2010). At high energies two temporal states are shown for 3C 264. The cyan upper limits at GeV energies and VHE correspond to the upper limits in 2017 from Fermi-LAT and VERITAS, while the dark blue data points and Fermi spectrum show the measurements from the 2018 enhanced state. We also show the contemporaneous optical and X-ray
fluxes from 2018 as dark blue circles; in the X-rays this includes the Chandra total measurement (fi lled point, no spectrum) and the VERITAS-concurrent Swift measurement (open point with butter
fly spectrum). The model shown (dashed and solid lines) is a self-consistent synchrotron + SSC model with parameters typical of BL Lac objects.
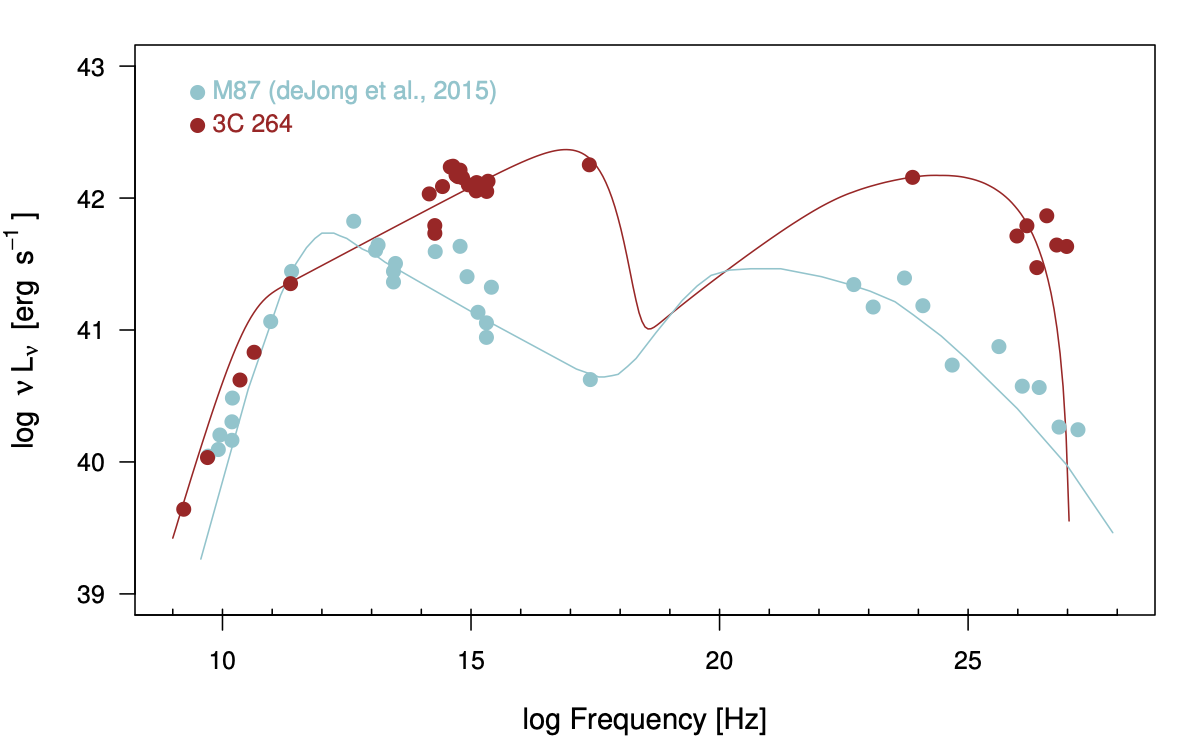
Figure 11: SED comparison for the 3C 264 non-thermal emission in 2018 (red) and M87 (light blue). The low-energy data points for both objects come from high-resolution imaging where the core flux can be isolated from other emission, while the HE (Fermi-LAT) and VHE data are for the total source. For M87, the data and fit are taken from the "average" state SED of de Jong et al. 2015, Figure 3. The model curve for 3C 264 is the same as in Figure 10. While both objects have remarkably consistent radio spectra, 3C 264 clearly has a much higher synchrotron peak, near or at the X-rays, where it is also nearly 50 times more luminous than M87. Similarly, the HE and VHE luminosity of 3C 264 is also clearly higher than M87.